Acute heart failure (AHF) is defined as the sudden presentation or sudden aggravation of signs and symptoms of heart failure, often requiring hospitalisation.1 It is a life-threatening condition, with in-hospital mortality ranging from 22% to 37% in severe cases of cardiogenic shock.2–4 Inotropes have been used in the management of patients with AHF for decades, especially for patients with systolic dysfunction – heart failure with reduced ejection fraction – due to their enhancing effect on cardiac contractility.3 They also have chronotropic and peripheral vascular effects that accompany their positive inotropic effect. They are most commonly used in hospital settings for patients with peripheral organ hypoperfusion and severely diminished cardiac output.5 However, the use of inotropes does have some adverse effects, including arrhythmogenesis and myocardial ischaemia, contributing to an unfavourable impact on long-term survival. As a result, their use is not recommended as routine practice for all patients with HF.1 However, they remain useful as short-term regimens for patients who present with AHF and evidence of hypoperfusion and impaired cardiac contractility. Careful selection of the most appropriate inotrope for each individual patient is of utmost importance (Table 1).
Traditional Inotropes
Currently available inotropic agents for the management of patients with AHF can fall into three categories, based on their mechanism of action: dopamine, dobutamine, norepinephrine and epinephrine that act as beta-agonists; milrinone, a phosphodiesterase (PDE) type 3 inhibitor; and levosimendan, a calcium sensitiser (Table 2).6,7
Beta-agonists
Dopamine
Dopamine is an endogenous catecholamine that exerts its dose-dependent effects on the cardiovascular system via its interaction with four different receptors: dopaminergic type 1 and type 2 and adrenergic alpha-1 and beta-1. When used at lower doses of up to 2.5 µg/kg/min, its primary net effect is vasodilation of the splanchnic, coronary and renal vasculature. While theoretically this effect seems favourable for the renal function of AHF patients, as it increases renal perfusion, there is no evidence that this translates to significant clinical benefit.
In a cohort of patients in intensive care with impending renal failure, the administration of low-dose IV dopamine did not prove to have any benefit in terms of reduction of peak creatinine levels or prevention of worsening renal function compared with placebo.8 Also, in the Dopamine in Acute Decompensated Heart Failure II (DAD-HF) trial published in 2014, the addition of low-dose dopamine to low-dose furosemide was not associated with improvements in symptoms, readmissions, mortality or renal function in patients with acutely decompensated chronic HF.9 Additionally, in the Renal Optimization Strategies Evaluation – Acute Heart Failure (ROSE-AHF) trial, adding low-dose dopamine or low-dose nesiritide to the standard diuretic regimen for patients with AHF and renal dysfunction did not lead to either decongestion or recovery of renal function.10
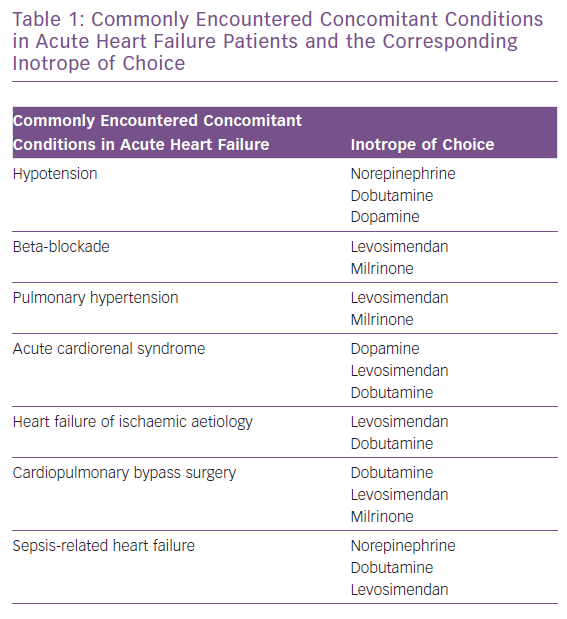
When administered in intermediate doses of 3–5 µg/kg/min, dopamine exhibits significant chronotropic and inotropic effects primarily by stimulating sarcolemmal beta-1 receptors in cardiomyocytes, but it also increases the pulmonary capillary wedge pressure (PCWP). When used at higher doses of more than 5 µg/kg/min, its net effect is a potent vasoconstriction, facilitated mostly via its effect on alpha-1 adrenergic receptors of the vasculature. This leads to a significantly elevated afterload that can prove detrimental for patients with AHF and systolic dysfunction. The most notable adverse effects of dopamine include hypertension and tachyarrhythmias that are more frequently encountered at doses of >10 µg/kg/min.5
Dobutamine
Dobutamine, a synthetic catecholamine, enhances cardiac contractility via its stimulatory effect on myocardial beta-1 receptors. It also affects the peripheral vasculature due to its combined action on vascular alpha-1 receptors and beta-2 receptors. In clinical practice, low doses of dobutamine (<5 µg/kg/min) for patients with AHF lead to increased cardiac output through enhanced inotropy, while simultaneously reducing afterload by exerting a vasodilatory effect on the peripheral arterial vasculature, thereby resulting in improved symptoms. However, several studies have linked its use with an increase in mortality rates. One meta-analysis showed that dobutamine was associated with higher risk of in-hospital mortality and future HF readmissions compared with the vasodilator nesiritide.11
Due to its potential for long-term complications, dobutamine is also used primarily in the in-hospital setting for short-term improvement of symptoms. It is noteworthy that in doses exceeding 5 µg/kg/min, the effect on peripheral vessels shifts towards vasoconstriction as the alpha-1 agonist effect becomes significantly more potent. The infusion rate of dobutamine ranges from 1–2 µg/kg/min up to 40 µg/kg/min. When prescribing dobutamine, it should be noted that the effect of dobutamine may be blunted in patients who are under chronic beta-blockade therapy, at least in usual doses. Another important limitation of dobutamine is that tolerance may develop even after short administration periods.12 In terms of adverse effects, dobutamine and has been proven to be arrhythmogenic in most dosage schemes, it has also been linked to the rare occurrence of eosinophilic myocarditis.13–15
Norepinephrine
Norepinephrine is an endogenous molecule, acting most potently on vascular alpha-1 adrenergic receptors, inducing vasoconstriction and increasing systolic and diastolic blood pressures. It also acts on cardiac beta-1 receptors, thereby exerting chronotropic and inotropic effects. Based on these properties, norepinephrine is primarily used in patients with AHF who present with cardiogenic shock, always in addition to another more potent inotropic agent. Norepinephrine is also used in combination with inodilators to prevent the development of hypotension.1
Norepinephrine is widely used for the management of other aetiologies, including septic shock. The Comparison of Dopamine and Norepinephrine in the Treatment of Shock (SOAP II) trial compared norepinephrine to dopamine as first-line agents for 1,679 patients with shock. Even though no statistically significant difference was found between the two arms in terms of mortality, dopamine was associated with an increased risk for adverse events including arrhythmias compared with norepinephrine. Notably, a subgroup analysis from the same trial including only patients with cardiogenic shock showed that norepinephrine was superior to dopamine in terms of reduction in mortality.16
In clinical practice, norepinephrine is usually infused at a rate of 0.01–0.03 µg/kg/min but can reach up to 1 µg/kg/min, until target blood pressure is achieved. Adverse events of norepinephrine include tachycardia that can significantly increase myocardial oxygen demand, which may be detrimental, especially in cases of active myocardial ischaemia. Also, it has been documented that norepinephrine has a direct toxic effect on cardiac cells, primarily due to cell apoptosis induced by beta adrenergic stimulation.17 Hypertension and tachyarrhythmias have also been reported with the use of norepinephrine.
Epinephrine
Epinephrine, also an endogenous catecholamine, exhibits dose-dependent effects. When administered at lower doses of up to 0.01 µg/kg/min, it primarily acts on beta-2 peripheral adrenergic receptors thereby causing vasodilation. However, when administered at an increased rate of >0.2 µg/kg/min, its effect on beta-1 and alpha-1 receptors predominates, resulting in overall positive inotropy and vasoconstriction. This vasoconstriction includes not only the peripheral vasculature but also pulmonary arterial and venous circulation.
Despite its inotropic, chronotropic and vasoconstrictive properties, epinephrine has been limited in everyday clinical practice to cases of cardiac arrest. This is due to the results from a pilot study by Levy et al. in 2011, in which epinephrine was compared with a regimen comprising of norepinephrine and dobutamine in patients with cardiogenic shock.18 The treatment strategies demonstrated comparable results in terms of haemodynamics; however, there were significantly increased rates of lactic acidosis, tachycardia, arrhythmia and gastric mucosal hypoperfusion observed in the epinephrine group, rendering it less safe to use in such patients.18
To further solidify these findings, the Cardiogenic Shock (CardShock) study looked at the trends and outcomes regarding common vasopressors and inotropes. It was found that epinephrine use in patients with cardiogenic shock was associated independently with increased 90-day mortality and with declining renal and cardiac function.19
Additionally, Leopold et al., in an individual-data-level meta-analysis, associated the use of epinephrine in the management of cardiogenic shock patients with a three times increased mortality rate compared with alternative drug regimens (OR 3.3; 95% CI [2.8–3.9]).20 Epinephrine is most commonly administered at an infusion rate ranging from 0.01–0.03 µg/kg/min to 0.50 µg/kg/min in refractory cases. Notable adverse effects of epinephrine include myocardial ischaemia, arrhythmias, hypertension, pulmonary congestion and intracranial bleeding.
Phosphodiesterase Type 3 Inhibitors
Milrinone
Milrinone, a PDE3 inhibitor, is a commonly used inotropic agent in patients with severe HF or cardiogenic shock.7 It inhibits PDE3, which physiologically degrades intracellular cyclic adenosine monophosphate (cAMP). Through this inhibition, cAMP accumulates in the cell, causing protein kinase A activation. This facilitates more calcium ions to enter the myocardial cell, thus potentiating the actin-myosin cross-bridging leading to increased cardiac contractility. This mechanism is independent of the beta-adrenergic pathway. As a result, the use of PDE3 inhibitors, and milrinone in particular, is suitable for patients with chronic HF under beta-blockade who present with AHF or cardiogenic shock compared with other inotropes.21
Another feature of the mechanism of action of milrinone is that the same intracellular processes is activated in smooth muscle cells of the peripheral and pulmonary vasculature, leading to a net vasodilatory effect in addition to its positive inotropic effect.22 This combination of actions classifies milrinone as an inodilator.
The short- and long-term effects of milrinone have been investigated. The Outcomes of a Prospective Trial of Intravenous Milrinone for Exacerbations of Chronic Heart Failure (OPTIME-CHF) evaluated the addition of IV milrinone on top of standard medical treatment in patients with AHF. No statistically significant benefit was found from the use of milrinone in terms of mortality or hospitalisations, whereas milrinone was linked to increased risk of prolonged hypotensive episodes and arrhythmias.23 In a subgroup analysis, milrinone was associated with increased mortality rates in patients with HF of ischaemic aetiology.24 Additionally, data from the Acute Decompensated Heart Failure National Registry (ADHERE) registry point towards the direction of increased mortality for dobutamine and milrinone compared with IV nitroglycerin or nesiritide.25
The Prospective Randomized Milrinone Survival Evaluation (PROMISE) trial concluded that the use of milrinone in symptomatic HF patients, despite optimal medical therapy, was associated with increased mortality and readmission rates compared with placebo.26 In clinical practice, milrinone is used in patients with AHF who maintain adequate systolic blood pressure (>85 mmHg). For patients with systolic blood pressure in the lower range (85–100 mmHg), milrinone is recommended to be used in combination with a vasoconstrictor, such as norepinephrine, to counteract its vasodilating effect. In addition, milrinone is preferred in patients who chronically receive beta-blockers, due to its beta-adrenergic pathway which is an independent mechanism of action.21 Due to its relatively long half-life and renal clearance, milrinone should be used with caution in patients with impaired renal function. Hypotension and tachyarrhythmias are also documented adverse effects of milrinone.23
Calcium Sensitisers
Levosimendan
Levosimendan exerts its effects by acting on troponin C, rendering the cardiomyocyte more sensitive to the already existing levels of intracellular calcium, thereby increasing its contractility. As its effects are not a result of influx of calcium in the myocyte, its arrhythmogenic potential is significantly limited. In addition to its positive inotropic effect, levosimendan leads to peripheral vasodilation via the opening of ATP-sensitive potassium channels on smooth muscle cells of the vasculature.27,28 Levosimendan has also been reported to have some PDE3 inhibitor properties and is also an inodilator.
Initial levosimendan studies showed promising results, despite their limited size. The Efficacy and Safety of Intravenous Levosimendan Compared with Dobutamine in Severe Low-Output Heart Failure (LIDO) study found that levosimendan was superior to dobutamine in terms of haemodynamic profile and mortality.29 Additionally, in the Safety and Efficacy of a Novel Calcium Sensitizer, Levosimendan, in Patients With Left Ventricular Failure Due to an Acute Myocardial Infarction (RUSSLAN) trial, levosimendan was associated with significantly decreased rates of death and deteriorating HF.30
However, two subsequent larger trials did not show positive results with levosimendan use. The 180-day mortality rate in the Levosimendan versus Dobutamine for Patients with Acute Decompensated Heart Failure (SURVIVE) trial was comparable in both arms and in the Randomized Multicenter Evaluation of Intravenous Levosimendan Efficacy (REVIVE-II) trial, despite a documented improvement in HF symptoms, levosimendan failed to prove beneficial in terms of mortality reduction and led to more cases of arrhythmias and hypotension.31,32
As a result, the use of levosimendan remains a topic of debate and it is only approved for use in Europe. Current European Society of Cardiology guidelines reserve its use in AHF patients with hypoperfusion that may be related to beta-blockade therapy.1 Levosimendan is usually administered at a rate of 0.05–0.20 µg/kg/min. Adverse effects include hypotension, AF, hypokalemia, headache and arrhythmias.
Novel Inotropic Agents
Omecamtiv Mecarbil
Omecamtiv mecarbil (OM) is the first and most investigated agent in a new class of inotropes called cardiac myosin activators.33 It exerts its effect by binding on an allosteric site on myosin itself. This leads to a stabilisation of the lever arm of myosin, rendering it primed. This effect when multiplied for numerous intracellular myosin molecules prior to the initiation of contraction, produces an increased number of primed myosin molecules and consequently an increased number of myosin heads available to cross-bridge with actin generating increased contractile force.34
It is important to note that the mechanism of action of OM is independent from calcium and cAMP, both of which contribute to arrhythmogenesis and myocardial ischaemia, as documented for traditional inotropes acting through these mediators. Additionally, since OM acts independently of the adrenergic pathway, it can be used as an alternative to milrinone and levosimendan in HF patients who are taking beta-blockers.35,36
The haemodynamic effects of OM have been tested in previous studies. Specifically, when compared to placebo, OM increased stroke volume and ejection fraction in AHF patients.37,38 These beneficial results initially failed to translate into clinically relevant results, as in a phase II trial patients treated with the agent did not report an improvement in dyspnoea.39 It should also be noted that an increase in troponin levels in patients treated with excessive doses of OM has been found. This has been hypothesised to be a result of diminished coronary filling during diastole due to prolonged ventricular systolic phase.39,40 However, at the regular therapeutically relevant exposures, no relationship was identified between troponin increases and systolic ejection time. The mechanism for the increases in troponin at therapeutically relevant exposures is currently unknown.
SERCA2a Modulation
Sarcoplasmic reticulum Ca2+-ATPase (SERCA), and its 2a isoform, is a sarcolemmal membrane-bound enzyme that handles free calcium influx back in the sarcoplasmic reticulum in the post-systolic period. SERCA2a, via this mechanism, affects the mechanics of both diastole and systole and its expression is long known to be reduced in HF patients, leading to systolic impairment.41,42 Consequently, it could be hypothesised that targeting the function and/or expression of this enzyme could be beneficial for HF patients.
SERCA2a Gene Therapy
SERCA2a modulation in HF patients can be achieved through gene therapy. An initial approach involved intracoronary administration of an adeno-associated virus type 1 encoding sarcoplasmic reticulum calcium ATPase (AAV1/SERCA2a). This gene therapy strategy initially proved to have an acceptable safety profile in and was tested in terms of efficacy in phase II trials.43 The Calcium Upregulation by Percutaneous Administration of Gene Therapy in Cardiac Disease (CUPID) trial included 39 patients with AHF in total. It was documented that gene delivery was superior to placebo in terms of symptomatic improvement, exercise tolerance, biomarkers and haemodynamic profile in the 6-month follow-up period.44 However, the larger CUPID II trial that followed, with 250 HF patients, could not replicate the results from the initial CUPID trial, as the administration of AAV1/SERCA2a did not significantly reduce HF-related endpoints, such as HF hospitalisations and worsening HF compared with placebo.45
Recommendations on the Optimal Administration of Inotropes in Acute Heart Failure
Approximately 10% of all hospitalised HF patients also have hypotension, decreased cardiac output and signs of peripheral hypoperfusion.25 In this considerable subgroup of patients, inotropes are recommended as part of their management (Figure 1).1 In refractory cases, circulatory support devices can be used in selected patients to maintain perfusion and haemodynamic stability for short periods of time.1 However, due to the sparsity of these devices compared with the large number of AHF patients, their use is reserved as bridge-to-transplant or other treatment decisions, while inotrope infusions as initial short-term support remain very common in everyday clinical practice.
The general principle that applies for the use of inotropes is to use them for the shortest amount of time possible and in the lower effective dose until the therapeutic goal of haemodynamic stabilisation (maintaining adequate BP and CO) and restoration of vital organ perfusion and function is achieved.
The types of AHF patients that usually receive inotropic support fall into two broad categories: those that present with cardiogenic shock and those with low BP and signs of hypoperfusion that do not present with overt cardiogenic shock. Patients presenting with cardiogenic shock have severely diminished cardiac output that leads to severe hypotension (below the 85 mmHg cut-off) and decreased peripheral and vital organ perfusion. This hypoperfusion becomes evident via clinical hallmarks of cardiogenic shock including cold extremities, elevation in lactate levels and reduced urine output and mentation changes.
The first step in treating a patient in cardiogenic shock should be aimed towards haemodynamically stabilising the patient and restoring tissue and vital organ perfusion. The regimen of choice includes the immediate administration of an inotropic agent, notably dobutamine, in combination with a vasopressor to offset the possible vasodilatory effect of the inotrope.1 In terms of the vasopressor agent of choice, data support the use of norepinephrine instead of epinephrine, since the latter has been repeatedly associated with worse mortality and renal outcomes, increased markers of hypoperfusion (lactate) and myocardial ischaemia (troponin).19 It should be noted that the combination of an inotrope and a vasopressor is also preferable to the use of a single vasopressor agent at an increased dose, since that would lead to a more potent vasoconstrictive effect, increasing cardiac afterload and the risk of ischaemia.46 In refractory cardiogenic shock cases, circulatory support with mechanical devices is recommended when feasible.
Inotropes are not only indicated for patients in overt cardiogenic shock (BP <85 mmHg), but also for HF patients with either marginal or even normal BP who do exhibit evidence of hypoperfusion. This evidence includes both clinical (cold extremities, altered mentation, diminished pulse pressure) and laboratory findings (increased blood urea nitrogen and creatine elevations in hepatic function tests and serum lactate and hyponatraemia). In these patients, the usual regimen of choice includes an inotrope or an inodilator with the goal to reverse the hypoperfusion. The decision to use which specific agent is individualised to each patient and dictated by the specific haemodynamic parameters of each case. Table 2 presents a summary of commonly encountered clinical scenarios and their corresponding inotropic agents.
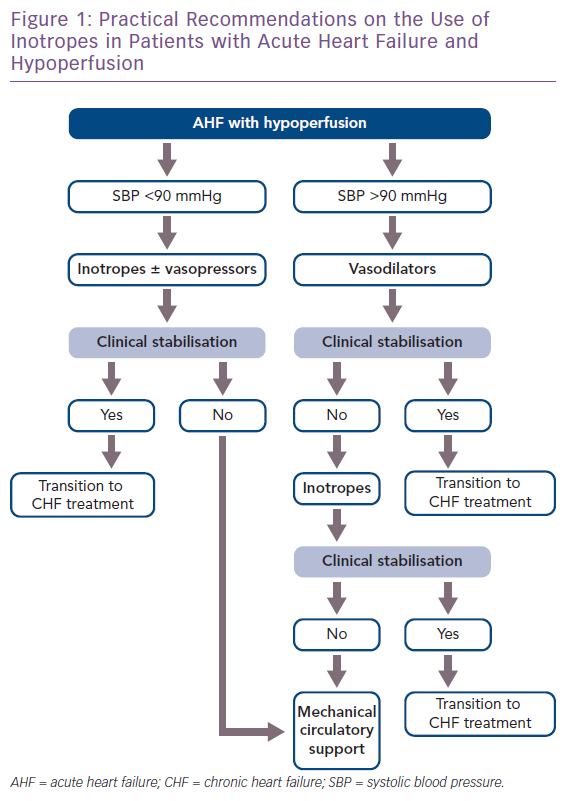
Inodilators (milrinone, levosimendan) possess some unique properties compared with other inotropic agents, which make them a more suitable therapeutic option for certain groups of patients. Due to their inherent vasodilatory effect, they are well suited for patients that have peripheral vasoconstriction. However, their use is not indicated if the systolic blood pressure is <90 mmHg. Additionally, if severe hypotension occurs after the administration of an inodilator, the concomitant use of a vasoconstrictive agent is recommended to counteract its vasodilatory effect. Also, since the inotropic effect of milrinone and levosimendan is independent of the beta-adrenergic pathway, they are the preferable option for the treatment of patients under chronic beta-blockade. It should be noted that in the Efficacy and Safety of Short‐term IV Treatment with Levosimendan vs Dobutamine in Decompensated HF Patients Treated with Beta‐blockers (BEAT-CHF) trial, the use of levosimendan and dobutamine were comparable in terms of haemodynamic improvement (PCWP decrease and increased cardiac output) after 1 day of therapy.47 A third and more specific group of patients who might benefit from the use of an inodilator over another inotrope are those with pulmonary arterial hypertension. Both milrinone and levosimendan may be indicated in these patients because of their documented vasodilatory effect on the pulmonary vasculature. Milrinone has been shown to reduce pulmonary vascular resistance in patients waiting for a heart transplantation.24
AHF patients commonly have concomitant renal and/or hepatic failure. In the case of primary renal failure, the choice of the appropriate inotropic agent is based primarily on its half-life. Dobutamine is the agent with the shortest half-life (2 minutes), whereas levosimendan has an 80-hour half-life. Therefore, dobutamine is the agent of choice for these patients. However, it should be noted that some data support the use of levosimendan in the subgroup of patients presenting with acute cardiorenal syndrome, as it increases renal perfusion more efficiently that other agents.48 For patients with impaired hepatic function, dobutamine is also the first choice as levosimendan is predominantly excreted via the liver. However, similarly to the cardiorenal syndrome indications, levosimendan has better supporting evidence in normalising liver function tests compared with dobutamine in people with acute cardio-hepatic dysfunction.49
For patients undergoing coronary artery bypass graft (CABG), the most widely used inotrope is dobutamine, as it provides better coronary perfusion without serious metabolic adverse effects.50 Additionally, milrinone has the disadvantage of being more arrhythmogenic than dobutamine while it may also counteract the vasoconstrive effect of the inhaled anaesthetics on the pulmonary vasculature.51,52 Finally, levosimendan has been shown to improve post-surgical outcomes, including length of stay and time to extubation.53,54 However, in the Levosimendan in Patients With Left Ventricular Systolic Dysfunction Undergoing Cardiac Surgery On Cardiopulmonary Bypass (LEVO-CTS) trial, the prophylactic use of levosimendan in isolated patients with reduced ejection fraction undergoing CABG failed to demonstrate benefit over placebo.55 When treating AHF that occurs after cardiotomy, the most widely accepted regimen comprises of dobutamine (or a different beta-agonist) in combination with milrinone or levosimendan. This combined approach is superior to monotherapy in terms of cardiac output, length of intubation and length of hospital stay.56
In sepsis-related AHF cases, recent data and recommendations suggest that dobutamine and norepinephrine should be the inotropic agents of choice.57,58 However, recent data concerning levosimendan use in these patients have been encouraging. Specifically, levosimendan has been shown to decrease serum lactate levels, exert a reno-protective role, and restore cardiac index without increasing myocardial oxygen demand leading to better short-term outcomes.59,60
Recommendations on the Optimal Weaning of Inotropes in Acute Heart Failure
As soon as congestion is alleviated and renal function improves, shown by an increase in urinary output and a decrease in blood urea nitrogen and creatinine levels, inotropic support should be tapered with the goal of complete weaning. During this time, standard oral HF treatment should be reinstated, and target doses should be reached after inotropic support is completely withdrawn. Unfortunately, there is a subset of patients who are unable to maintain adequate BP and perfusion without inotropic support despite multiple attempts to discontinue them. These patients, often referred to as inotrope-dependent, are usually supported with inotropes for prolonged periods of time either as a bridge (to transplant or a left ventricular assist device) or as part of a broader palliative strategy aimed at symptomatic relief.
Conclusion
Inotropic agents have long been associated with adverse events including arrhythmogenesis and unfavourable long-term mortality outcomes. However, they remain a key weapon in the arsenal of physicians that manage AHF patients, due to the lack of other efficacious medical or interventional strategies. Their use should be limited to the minimum possible dose for the shortest amount of time adequate to restore BP and peripheral perfusion. Ongoing and future research in the field of inotropes aims to assess the safety and efficacy of new molecules that act through novel or alternative pathways to reduce the adverse events profile of inotropes and reduce their negative effect on long-term survival.