Approximately 90% of the more than 1 million yearly heart failure hospitalisations in the US and Europe are a result of symptoms and signs of fluid overload and are associated with readmission rates of 24% and 50% at 30 days and 6 months, respectively.1,2 Recurrent heart failure-related hospitalisations have uniformly been associated with worse outcomes, independent of age and renal function.3
Abnormal fluid handling begins in the asymptomatic stages of heart failure and leads to physiological abnormalities in multiple organ systems.4–9 Elevations of central venous pressure are rapidly transmitted to the renal veins, causing increased interstitial and tubular hydrostatic pressures, which decrease net glomerular filtration in both acute and chronic heart failure.8,10–12 The consistent finding that inadequate reduction of fluid excess in acute heart failure patients trumps increases in serum creatinine in predicting poor outcomes underscores the importance of effective decongestion. If a decrease in intravascular volume by fluid removal causes small transient increases in serum creatinine, achieving euvolaemia may still be essential to protect the kidneys in the long term.13,14
Diuretic Resistance in Heart Failure
Diuretics, the most widely used drugs to reduce fluid excess, become increasingly ineffective with heart failure progression as a result of impaired absorption, decreased renal blood flow, azotaemia and proteinuria, all resulting in reduced levels of active diuretics in the tubular lumen.15,16 Several definitions of diuretic resistance and responsiveness have been proposed.16
The clinical hallmarks of diuretic resistance are insufficient symptom relief, higher risk of in-hospital worsening of heart failure, increased mortality after discharge and a threefold increase in rehospitalisation rates.16 Among more than 50,000 patients enrolled in the Acute Decompensated Heart Failure National Registry (ADHERE) and treated with conventional diuretic therapy, only 33% lost 2.3 kg or more, 16% gained weight during hospitalisation and almost 50% were discharged with unresolved congestion.17 Irrespective of diuretic strategy, 42% of acute heart failure subjects in the Diuretic Optimization Strategies Evaluation (DOSE) trial reached the composite endpoint of death, rehospitalisation, or A&E visit at 60 days.18 All other pharmacological approaches studied as adjuncts or alternative therapies for acute heart failure have failed to improve long-term outcomes.19–21 Therefore, alternative and more effective methods of fluid removal are critically needed. One promising therapy is extracorporeal ultrafiltration (UF).22 Greater access to UF has been facilitated by the developmentof simplified devices that do not require specialised technicians or acute care settings.22
Process of Fluid Removal by Ultrafiltration, Haemofilters, Pumps and Vascular Access
UF consists of the production of plasma water from whole blood across a semipermeable membrane (haemofilter) in response to a transmembrane pressure gradient.23 The newer, simplified UF devices afford the advantages of small size, portability, low blood flow rates and an extracorporeal blood volume below 50 ml.24 They can provide a wide range of UF rates (0–500 ml/h) and do not mandate admission to intensive care units. Vascular access can be achieved through either a peripheral or a small central vein such as the internal jugular or subclavian veins. The characteristics of two of these devices are shown in Figure 1.
Differences in Fluid Removal with Ultrafiltration Versus Diuretics
Loop diuretics selectively block the Na+/K+/2Cl− cotransporter in the luminal membrane of the medullary thick ascending loop of Henle. This builds a concentration gradient towards the renal papilla, which is essential to concentrate the urine. This is why loop diuretics lead to the production of hypotonic urine.23
In contrast, because the ultrafiltrate is almost isotonic with plasma, approximately 134–138 mmol of sodium are removed with each litre of ultrafiltrate.23 Thus, for any amount of fluid withdrawn, more sodium is likely to be removed with UF than with diuretics.24,25 Furthermore, changes in intravascular volume in response to diuretics are unpredictable.8,23,26 As opposed to loop diuretics-induced renin release by the afferent arteriole, neurohormonal activation only occurs with UF if fluid removal exceeds the plasma refilling rate, causing intravascular volume depletion (Table 1).25,27,28 Plasma water movement from the interstitium to the vasculature varies between patients according to serum albumin concentration, that is, serum oncotic pressure and capillary permeability. Although rates of UF ≤250 ml/h are less likely to exceed the plasma-refilling rate, the pace of fluid removal should be adjusted to patients’ vital signs, serum creatinine and urine output to preserve blood volume and haemodynamic stability (Figure 2).27,28
Randomised Controlled Trials of Ultrafiltration
The fact that refill of the intravascular space from the oedematous interstitium decreases as fluid is removed led to the hypothesis of the Ultrafiltration Versus Intravenous Diuretics for Patients Hospitalised for Acute Decompensated Heart Failure (UNLOAD) trial that initiation of UF before diuretic administration might be superior to IV loop diuretics in fluid overloaded heart failure patients.29 Compared with standard care, the UF group had greater weight loss and similar improvement in dyspnoea score (the co-primary endpoints) at 48 hours. The percentage of patients with increases in serum creatinine levels of 0.3 mg/dl or more was similar in the UF and control groups up to 90 days.29 There was no between-group difference in the duration of the index hospitalisation. In UNLOAD, 90-day heart failure events were a pre-specified secondary endpoint, and the investigators determined whether these were related to worsening heart failure or not. Because UNLOAD did not have an independent clinical event committee to adjudicate whether an event was heart failure-related or not, the possibility of patient or investigator bias cannot be excluded. Nevertheless, compared with standard care, at 90 days patients treated with UF had 52% fewer unscheduled visits, 44% fewer heart failure-related rehospitalisations and a 63% reduction in rehospitalisation days. Limitations of the UNLOAD trial include lack of treatment targets, blood volume assessments, cost analysis and adjudication of events by an independent clinical event committee.
In the Effects of ULTRAfiltration vs DIureticS on clinical, biohumoral and haemodynamic variables in patients with deCOmpensated heart failure (ULTRADISCO) study, at 36 hours, UF patients had greater reduction in body weight, signs and symptoms of heart failure, aldosterone and N-terminal pro B-type natriuretic peptide levels, and systemic vascular resistance, as well as greater improvements in cardiac performance, compared with the diuretic group.30
The Cardiorenal Rescue Study in Acute Decompensated Heart Failure (CARRESS-HF) compared the effects of UF, intended to be delivered at a fixed rate of 200 ml/h, with those of stepped pharmacological therapy (consisting of adjustable doses of IV loop diuretics, thiazide diuretics, vasodilators and inotropes) in acute heart failure patients with pre-randomisation increases in serum creatinine.31,32 The primary endpoint of CARRESS-HF was the bivariate change in serum creatinine and body weight from baseline to 96 hours after randomisation.31,32 According to the CARRESS-HF design manuscript, this primary endpoint assumes that weight loss is a measure of effective fluid removal and that an increase in serum creatinine represents acute tubular injury.30 In CARRESS-HF, both groups lost a similar amount of weight but increases in serum creatinine were greater with UF.32 In addition, a higher percentage of patients in the UF group experienced serious adverse events.32 However, the fact that 37 patients (39%) randomised to the UF group received only diuretics, or were given these drugs before the assessment of the primary endpoint at 96 hours, impairs adjudication of adverse events to one or the other therapy.32
In heart failure, increases in serum creatinine (0.3 mg/dl or more) have been equated to actual renal tubular damage, which portend adverse long-term prognosis, but transient increases in serum creatinine can merely reflect a haemodynamically-driven decrease in estimated glomerular filtration rate (eGFR) similar to that occurring with angiotensin converting enzyme inhibitors. Notably, recent studies show that transient increases in serum creatinine may indicate more complete decongestion and are associated with better post-discharge outcomes.4,14,33
The per-protocol analysis of CARRESS-HF was recently published.34 With the inclusion of only subjects who received their randomised treatment, UF was associated with higher cumulative fluid loss, net fluid loss and relative reduction in weight compared with stepped pharmacological therapy. The UF group had higher serum creatinine and blood urea nitrogen by 72 hours.34 The per-protocol analysis of CARRESS-HF also confirms the astonishing finding of the primary publication that 90% of the study’s subjects had unresolved congestion at the time of evaluation of the primary endpoint.32 It remains difficult to discern whether the inability to achieve adequate decongestion was a result of intrinsic ineffectiveness of the CARRESS-HF’s stepped pharmacological therapy strategy, or of inadequate adherence of the investigators to the study’s protocol. In contrast, causes for the poor performance of UF, which could be surmised in CARRESS-HF’s intention-to-treat analysis, are laid bare by the per-protocol analysis. At 96 hours – the time of evaluation of the primary endpoint – only 30 of 94 subjects (32%) were still undergoing UF, compared with the stepped pharmacological therapy arm, in which 76 of 94 (80%) remained on treatment. For 28 of 64 patients (43%) no longer on UF by 96 hours, the reason for therapy discontinuation is described as “MD decision”. Because such choice is separate from the other listed causes of therapy discontinuation, including achievement of optimal volume status, haemodynamic instability, evidence of volume depletion, increased serum creatinine, filter clotting and vascular access failure, the suspicion arises that UF was prematurely terminated either for convenience, lack of familiarity with the therapy, or both.34
The unprecedented incidence of filter clotting, which occurred in 23 of 64 patients (36%) no longer on UF at 96 hours is also disconcerting. The estimated UF rates were 83 (46–109) ml/h; 140 (83–178) ml/h; 107 (32–178) ml/h and 70 (21–115) ml/h for each sequential 24-hour period from baseline to 96 hours. Low UF rates should reduce, rather than increase, filter clotting by decreasing filtration fraction.35 Therefore, the most plausible culprit of filter clotting was the use of excessively low blood flows, which augment the risk of haemofilter clotting by increasing the filtration fraction. Notably, UF rates at 48 and 72 hours were higher than those used at 24 hours. This fact suggests disregard for the physiological principles of fluid shift between the interstitium and the vascular space described by Starling.36
In the UNLOAD trial UF, used at rates higher than those reported for CARRESS-HF, achieved better decongestion compared with pharmacological therapy, without greater increases in serum creatinine.29 This apparent paradox can be explained by considering that in CARRESS-HF, by protocol, UF rates were not tailored to individual patients baseline renal function, vital signs and urine output. The low average UF rates in CARRESS-HF may be misleading as they may not reflect either lingering congestion in the subjects who might have needed more fluid extraction or overzealous fluid removal in individuals at higher risk of hypovolaemia.32,34
With awareness of the inherent limitations of any per-protocol analysis, the results of the CARRESS-HF per-protocol analysis differ significantly from those of the intent-to-treat analysis and corroborate the key findings of UNLOAD, which demonstrated more effective decongestion with UF than with pharmacological therapies.29 However, in contrast to UNLOAD, which showed greater reductions in heart failure events in the UF arm compared with the diuretic arm, the per-protocol analysis of CARRESS-HF showed no differences in 60-day outcomes between the UF and pharmacological arms.29,32,34 This finding is hardly surprising because 90% of the CARRESS-HF population was not adequately decongested when the primary endpoint was evaluated.
In the Continuous Ultrafiltration for Congestive Heart Failure (CUORE) trial, 27 UF-treated patients had fewer heart failure-related rehospitalisations during one-year follow up than the 29 standard care subjects, despite similar weight loss at discharge.37 In CUORE, diuretics were continued during UF because of the belief that this strategy may increase sensitivity to diuretics by augmenting urinary sodium excretion.37 The wisdom of removing the ‘diuretic holiday’, during which loop diuretic-induced neurohormonal activation does not occur, is controversial.29,38,39
The Aquapheresis versus Intravenous Diuretics and Hospitalization for Heart Failure (AVOID-HF) trial tested the hypothesis that patients hospitalised for heart failure treated with adjustable UF would have a longer time to first heart failure event within 90 days than those receiving adjustable IV loop diuretics.40
The AVOID-HF trial, designed as a multicentre, one-to-one randomised study of 810 hospitalised heart failure patients, was terminated unilaterally and prematurely by the sponsor (Baxter International) after enrolment of 224 patients (27.5%). Both therapies were adjusted according to patients’ vital signs, renal function and urine output (Figures 3 and 4).41 Patients in the adjustable UF group had a longer time to first heart failure event than patients in the adjustable diuretics group (62 versus 34 days; p=0.106), but this difference was not statistically significant. However, there was enough evidence to show that within 30 days after discharge patients in the adjustable UF group had fewer heart failure and cardiovascular events than the adjustable diuretics group.40
Importantly, these events were adjudicated by an independent committee blinded to randomised therapy.40 The finding of similar renal function changes in the two groups are consistent with that of UNLOAD.29,40 In AVOID-HF, the average UF rate of 138 ml/h and therapy was delivered over an average of 70 hours.40 Adjustments of UF rates to individual patients’ haemodynamics and renal function may explain the lack of difference in serum creatinine between groups, despite a larger net fluid loss with UF.40 Restoration of diuretic responsiveness may be a key mechanism by which UF delays recurrence of heart failure events.40
Significantly more patients in the UF group than in the diuretics group (31% versus 17%; p=0.018) experienced adverse events of special interest (infection requiring IV antibiotics, bleeding requiring transfusion, symptomatic hypotension requiring vasopressor agents or rapid fluid replacement, drop in haemoglobin greater than 3 g/dl and acute coronary syndrome requiring intervention). Serious therapy-related adverse events occurred at higher rates in the UF group than in the diuretics group (14.6% versus 5.4%; p=0.026).40 In AVOID-HF, UF-related adverse events were fewer than in CARRESS-HF, but the excess of therapy-related complications with UF is a serious concern.32,40 Further study of the specifics of providing UF is needed to identify strategies aimed at minimising access-related and other potentially preventable complications.32,40
Knowledge Gaps in the Use of Ultrafiltration in Acute Heart Failure
Selection of Potential Candidates
Patient selection and fluid removal targets for UF remain incompletely understood.32,40 Current practice guidelines recommend the use of UF only for patients with a degree of diuretic resistance similar to that of CARRESS-HF subjects.32,42,43 In AVOID-HF, fine-tuning of UF rates in response to vital signs, renal function or urine output resulted in greater net fluid loss and was associated with fewer 30-day heart failure events, without a greater increase in serum creatinine levels, compared with the adjustable diuretic group.40 These observations underscore the critical need for additional investigation of UF as both first-line and rescue therapy, provided that UF rates are adjusted in each patient in response to changes in vital signs and renal function.32,40
As a result of the potential complications and cost of UF, it should not be used in all patients with acute heart failure. For example, patients with de novo heart failure or those not receiving daily diuretics are likely to respond to IV diuretics. The lingering question is, which patients who develop acute heart failure despite daily oral diuretics should receive UF instead of IV diuretics? To date, all studies of UF in heart failure patients have used only clinical signs and symptoms of congestion, both as inclusion criteria and fluid removal targets. This is challenging, given the poor correlation between clinical signs and objective measures of increased filling pressures.44
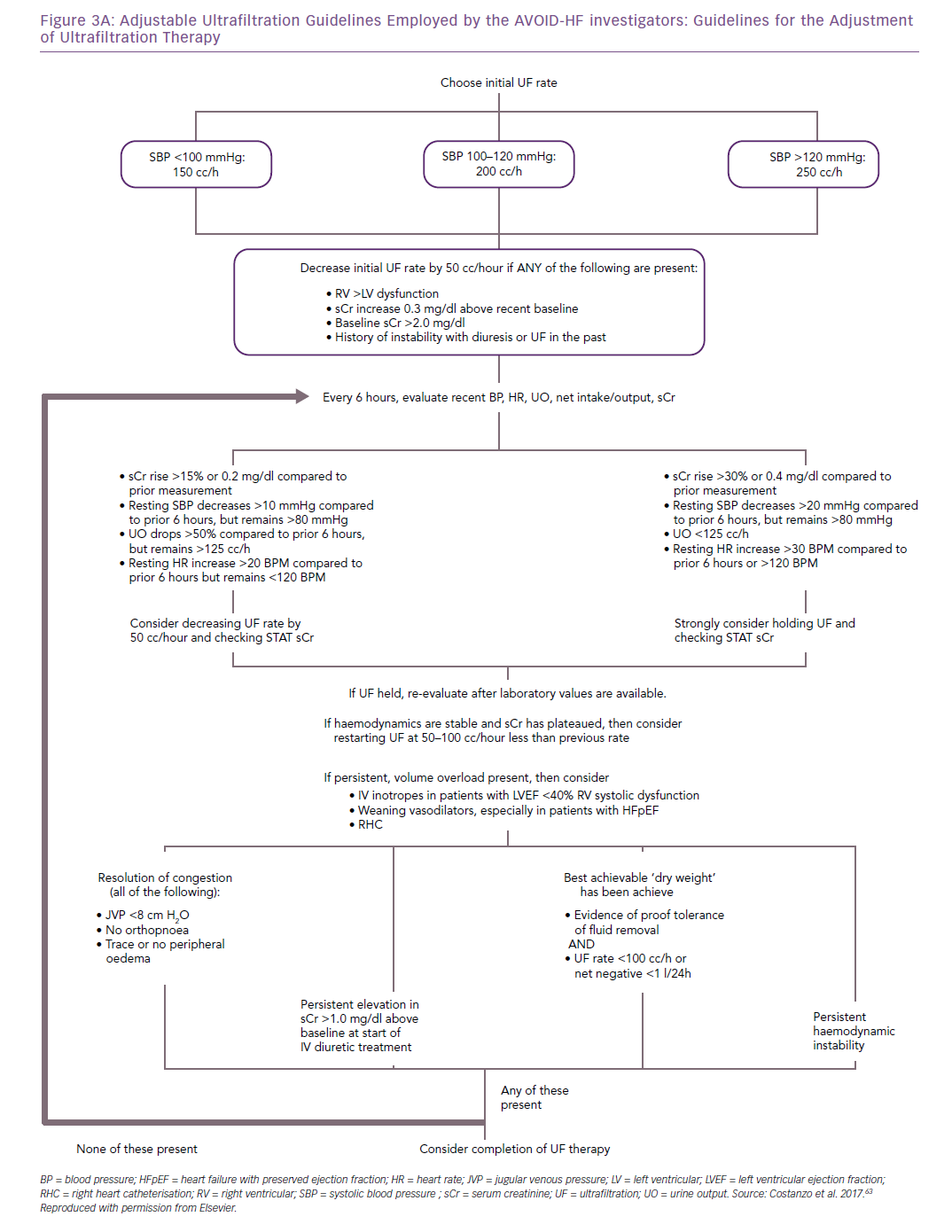
The hypothesis that UF may be especially effective in patients with urinary sodium concentrations <100 mEq after a specified dose of IV diuretics should be tested in randomised trials. A single non-randomised prospective cohort study showed similar effects of UF in heart failure with reduced versus preserved left ventricular ejection fraction.45 However, because these two heart failure types are pathophysiologically and clinically different, response to UF in the two heart failure settings should be evaluated in controlled trials.
Fluid Removal Targets and Monitoring of Ultrafiltration Therapy
An important general recommendation is that once an initial UF rate is decided it should be either maintained or reduced, because capillary refill from the interstitium decreases as fluid is removed.27 While optimal rate and duration of UF must be individualised, UF rates >250 ml/h are not typically recommended.40,41 Patients with predominantly right sided, or preserved ejection fraction heart failure are more susceptible to intravascular volume depletion and may only tolerate UF rates ≤100 ml/h.46 Clinical experience suggests that UF is better tolerated when conducted with low fluid removal rates over prolonged periods of time.32,40
Typically, patients’ current weight is compared with the weight recorded in the absence of congestive signs and symptoms and this ‘dry weight’ is used as the target for fluid removal. Given the detrimental renal effects of increased central venous pressure,8,11,12,27,28,47 controlled studies may evaluate whether fluid removal by UF should be aimed at achieving certain central venous pressure targets, i.e. ≤10 mmHg. However, central venous pressure is poorly correlated with measured blood volume and, in the context of UF, fluid overload may still be present despite achievement of a low cardiac filling pressure.48 Instead of invasive haemodynamic measurements, ultrasonography can help estimate central venous pressure with evaluation of the respiratory excursions of the diameter of the inferior vena cava.49 However, the reliability of ultrasonography strictly depends on operator’s skill and patient’s respiratory effort.49
Baseline pulmonary artery diastolic pressure consistently predicts heart failure events. Interventions aimed at reducing pulmonary artery pressures to pre-specified target ranges have effectively reduced heart failure events without significant renal function changes.50,51
The CardioMEMS™ HF System (Abbott) measures pulmonary artery pressures as frequently as clinically indicated. In patients implanted with the CardioMEMS device, it is conceivable that fluid can be removed by UF until achievement of the target range of pulmonary artery pressures that effectively reduced heart failure events.50,51
Blood Volume and Fluid Excess Assessment
The haematocrit is the ratio of the volume occupied by red blood cells to that of the whole blood. Since red blood cell mass does not change in the short term, unless bleeding occurs, fluctuations in haematocrit reflect changes in intravascular volume.52
Online haematocrit sensors permit continuous estimation of blood volume changes during UF and can be programmed to stop fluid removal if the haematocrit exceeds a threshold set by the clinician; for example, 5–7%, and resume therapy when the haematocrit value falls below the pre-specified limit, indicating an adequate refilling of the intravascular volume from the interstitial space.52 However, because numerous factors, such as change in body position, can alter haematocrit values, physical, laboratory and haemodynamic variables should be concomitantly assessed to determine the appropriate UF rates and the amount of fluid that should be removed.52
Bioimpedance vector analysis is based on the principle that whole-body impedance to an alternating current reflects total body water (r=0.996).53 Measurements of bioimpedance vector analysis require two pairs of electrodes placed on the wrist and ankles and the application of a 50 kHz alternating microcurrent (CardioEFG, EFG Diagnostics).53 Bioimpedance vector analysis could be used to determine fluid status before treatment initiation and serially to guide the amount and rate of fluid removal. Diaphoresis, hirsutism, incorrect electrode placement, cutaneous alterations, or improper electrical grounding can affect the reliability of bioimpedance vector analysis measurements. Bioimpedance spectroscopy is also being investigated in patients with heart failure.54 Unfortunately, no existing bioimpedance-based method can differentiate intravascular from interstitial extracellular fluid volume, a distinction that is critical for safe and effective fluid removal.53,54
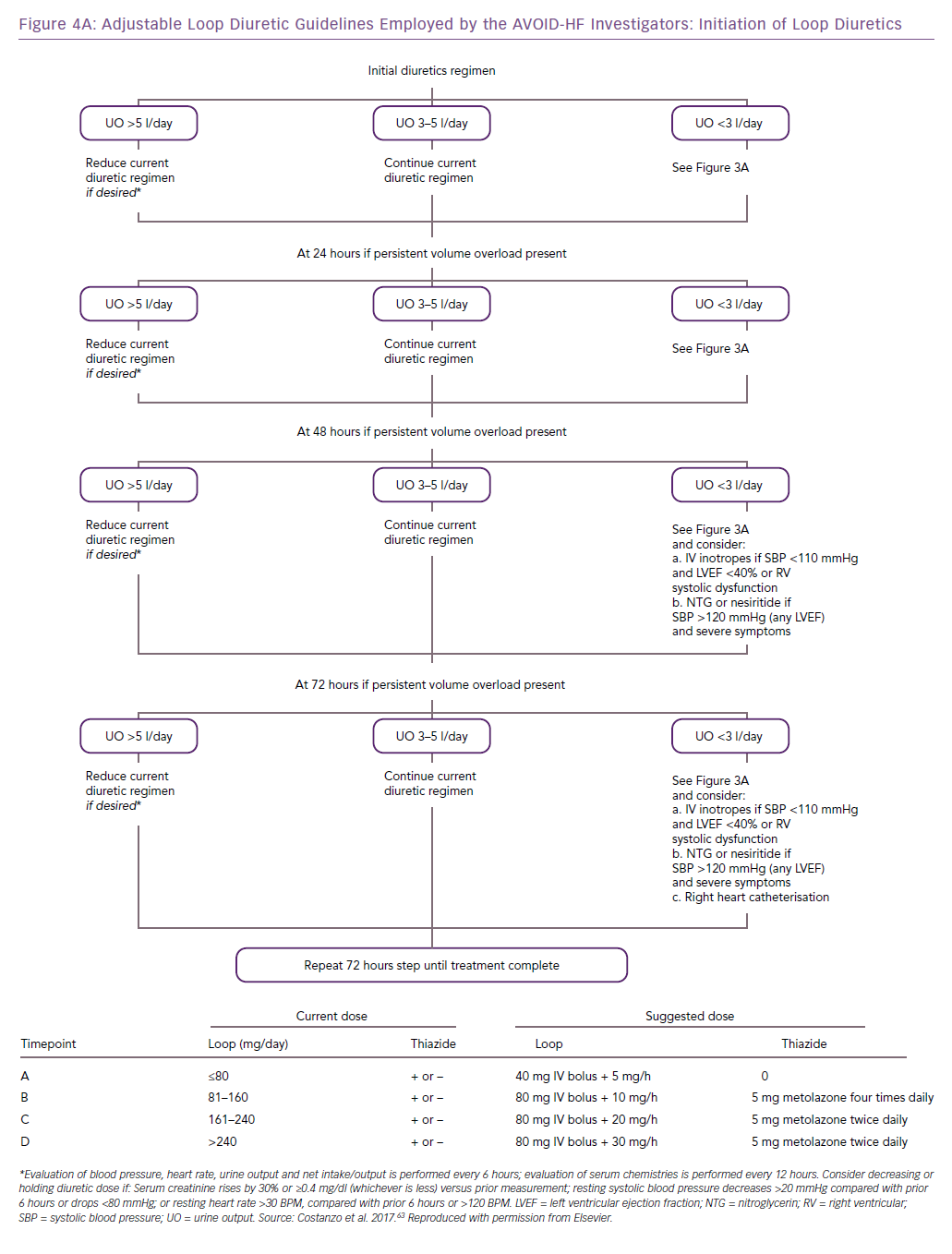
The measurement of blood volume using albumin labelled with iodine-131 is accurate, but the six to nine blood draws needed to create the dilution curve make it impractical for the serial assessments needed during fluid removal.55 The lack of optimal methods for the estimation of blood volume and fluid excess underscores the critical need for research in this area.
Biomarkers
The use of natriuretic peptides to assess volume status and guide decongestive therapies cannot be recommended because fluid overload is not the sole cause of increases in the levels of these biomarkers.56 The removal of fluid to achieve pre-specified natriuretic peptide levels is untested in acute heart failure. Serum creatinine is used to guide fluid removal because of the assumption that its level indicates both renal filtration and tubular status. However, serum creatinine was established and validated as a measure of renal function only at the point of steady state. Therefore, it is puzzling that serum creatinine is considered an accurate measure of renal function in patients with acute heart failure, where the rates of creatinine production and excretion can be altered.
Screening of 3.8 million patients revealed that 75% of serum creatinine elevations are a physiologically appropriate response to decreases in intravascular fluid volume and do not reflect acute renal damage.57 More importantly, serum creatinine can be normal with true tubular injury because measurable changes are delayed.58 Typically, haemodynamically-driven increases in serum creatinine can be reversed with treatment in 24 to 72 hours, whereas changes as a result of acute tubular damage may persist for weeks.59 As such, the duration of serum creatinine increase is more predictive of outcomes than the magnitude of this biomarker’s elevation.59 Indeed, the use of increases in serum creatinine as an endpoint for acute heart failure trials has been questioned. In DOSE, increase in subjects’ serum creatinine from baseline to 72 hours was associated with lower risk for the composite outcome of death or heart failure events. In contrast, there was a strong correlation between improved renal function during hospitalisation and poor 60-day outcomes.60
Neutrophil gelatinase-associated lipocalin (NGAL) is secreted in the urine and the plasma by a damaged kidney within 3 hours of pathogenic events such as sepsis, nephrotoxins, obstruction or ischaemia. Furthermore, the amount of secreted NGAL (from 20 ng/ml up to 5 μg/ml) was proportional to the severity and time to resolution of the causative event.58 Evidence suggests NGAL is not expressed when serum creatinine increases as a result of volume depletion.61 In future, urinary levels of NGAL and other biomarkers of tubular injury may help to distinguish a rise in serum creatinine as a result of a haemodynamically mediated decrease in eGFR versus true tubular injury.57
Suggestions for Future Studies of Ultrafiltration
Priority should be given to mechanistic studies, including evaluation of diuretic responsiveness at baseline, during and after fluid removal using the measures described in this article.16 Haemodynamic measurements that reflect fluid status, for example central venous pressure and pulmonary artery diastolic pressure, should also be performed at baseline and throughout therapy.
Specific haemodynamic targets indicative of optimal fluid status should be established in individual patients, similar to the strategies used to guide medication adjustment in studies of pulmonary artery pressure sensors.50,51 Different UF rates should be tested in terms of their ability to reach these haemodynamic targets without causing renal tubular damage, as detectable by increase in urine levels of biomarkers such as NGAL.57,58,61,62 This will require simultaneous measurement of the selected haemodynamic values and biomarker levels capable of differentiating rises in serum creatinine as a result of decreases in eGFR produced by intravascular fluid removal, from those reflective of renal injury.
Serial measurements of urine and ultrafiltrate sodium content (non-invasive, inexpensive and readily available) may also help to better characterise and compare the amount and pattern of sodium extraction during UF therapy and conventional diuretic-based regimens. The results of mechanistic studies are essential to determine how fluid removal rates and amounts should be adjusted in individual subjects of future controlled trials, that is, ‘precision’ fluid removal.
Development of vascular accesses and UF device components that increase the efficiency and safety of the therapy is also critical. The device- and therapy-related adverse events observed in previous trials should undergo careful re-evaluation to determine which were preventable or related to operator experience versus those that were inherent to how therapy was delivered or were unpredictable.32,40
Only after these issues have been satisfactorily addressed should a carefully designed, adequately powered study be considered to prospectively compare UF with pharmacological fluid removal therapies. All treatments should be tailored to individual patients’ haemodynamic and renal status. In addition, the study’s follow-up period should be long enough to permit the evaluation of morbidity (rehospitalisations) and mortality. Future trials should evaluate whether higher costs of mechanical fluid removal during the index hospitalisation are offset by the savings resulting from fewer heart failure events in UF-treated patients.
As the cost of in-patient care is high, serious consideration should be given to studies in the out-patient setting to determine the relative safety and effectiveness of intermittent pharmacological and mechanical fluid removal therapies for the prevention of heart failure hospitalisations. Intermittent out-patient UF to restore responsiveness to oral diuretics is also a strategy that deserves investigation. Finally, technological advances may permit the development of wearable UF devices capable of delivering individualised UF therapy.
UF is an attractive alternative therapy because it predictably removes total body sodium. Practice guidelines recommend extracorporeal fluid removal by UF only in diuretic-resistant fluid-overloaded heart failure patients. In future studies, UF should be adjusted according to the individual’s haemodynamic and renal profiles, and patient selection, fluid removal amount, duration and rate should be guided by objective, complementary and informative measures of fluid overload and kidney function. The urgency of these investigations is underscored by the alarming prognostic and economic implications of recurrent heart failure hospitalisations, which remain unacceptably high with conventional pharmacological therapies.